The magnetoelastic pseudobinary Laves phase compounds Hf1-xTaxFe2, crystallizing in the geometrically frustrated hexagonal MgZn2-type structure. Neutron powder diffraction (NPD) on Hf0.86Ta0.14Fe2 was collected from 200 to 400 K. To completely determine the magnetic structures, we investigate the diffraction data at very low Q. There are two FM arrangements allowed: one with moments pointing in the ab plane and another with moments along the c axis. The latter is excluded and the in-plane model is able to reproduce these features of NPD. Since the collinear AFM structures were excluded by NPD, only the frustrated AFM structures can be considered where magnetic moments of Fe at the 6h sites form the 120? structure in the ab plane and interlayer coupling can be either FM or AFM. The (102) and (111) peaks can be obtained only for the interlayer AFM configuration.
At Tt of 255 K, a exhibits a large jump, ¦¤a/a¡Ö0.3%, and the system transforms simultaneously to the FM state. Below Tt , a stays nearly constant, while c gradually decreases. As shown by the slope triangle, V is increased by around 0.5% with varying temperature from 260 to 220 K, which is translated into a tremendous coefficient of volumetric thermal expansion, -123 ¡Á 10-6/K.
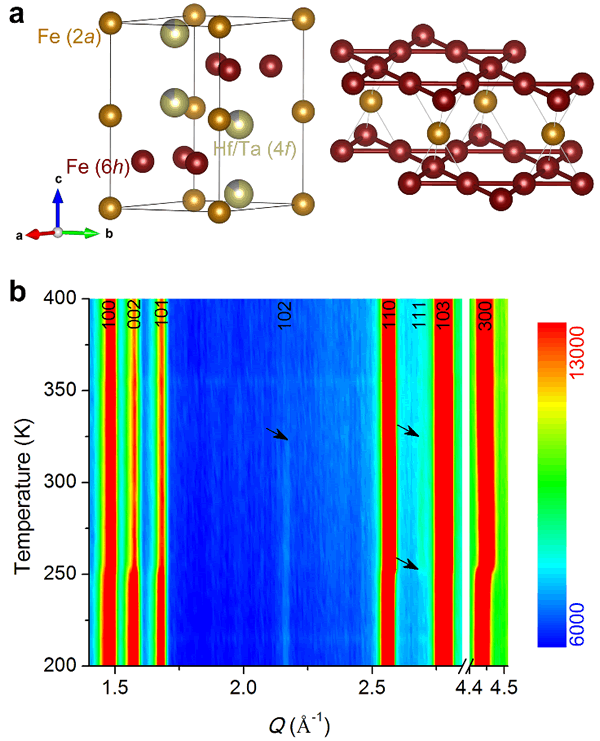
Fig. 4 (a) The crystal structure of Hf1-xTaxFe2. (b) The contour plot of neutron diffraction intensity. The features are pointed out by the arrows.
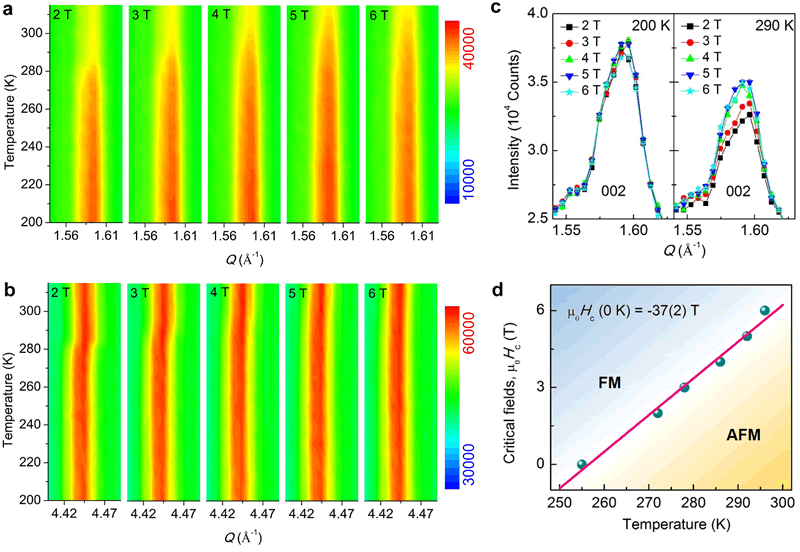
Fig. (a) and (b) The contour plots of diffraction intensity of Bragg peaks (002) and (300) at different magnetic fields, respectively. (c) The Bragg peak (002) at 200 (left) and 290 K (right) as a function of magnetic fields, respectively. (d) The phase diagram of the first-order transition. The phase boundary is well described by a linear relation with intercept of -37(2) T.
The magnetic phase competition is examined in the magnetic fields and temperature phase space by employing in situ neutron diffraction. The contour plots of the diffraction intensity of the (002) and (300) Bragg peaks are shown in Figs. 4(a) and 4(b), respectively. The crossover of the intensity of the (002) peak, an indication of AFM to FM transition, is prompted to higher temperatures with increasing magnetic fields. This means the AFM state is suppressed, in accordance with previous specific-heat values measured with magnetic fields and our diffraction data under applied magnetic fields. At 200 K, it is FM, so that the intensity is much less susceptible to magnetic fields than at 290 K where it is AFM. The intensity at 290 K becomes almost field independent beyond 4 T, which roughly defines the critical field inducing the AFM to FM transition. In response to the applied magnetic fields, the lattice is concomitantly expanded in the ab plane, as seen in the shift of the (300) peak. The detailed field dependencies of lattice dimensions are compared with their temperature dependencies. The diffraction under in situ magnetic fields enables us to directly determine the magnetic phase diagram. The transition temperature is defined as one where the lattice constant a of the FM state starts to sharply drop. There is a linear relationship, depicted by the fitting shown in Fig. ?(d), similar to the other systems with AFM to FM transitions. The intercept represents the extrapolated critical fields for the AFM to FM transition at 0 K.
|